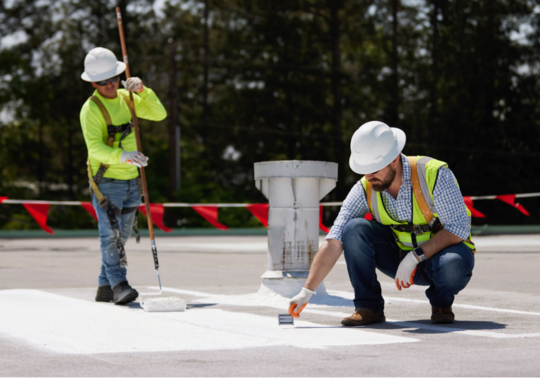
In Your Community
Ventajas de los revestimientos de silicona para techos
Como contratista comercial de construcción de techos, usted es responsable de elegir los materiales adecuados para cada trabajo. Con tantas opciones disponibles, tomar una decisión puede resultar una tarea difícil.Cada vez más, los profesionales del sector están optando por los revestimientos de silicona para techos por su fuerza y durabilidad. Este tipo de revestimientos sirve para extender la vida útil de un techo con una estructura firme y, posiblemente, para que los propietarios ahorren tiempo y dinero al retrasar la reparación completa del techo. Además, sus propiedades de restauración funcionan a la perfección con la mayoría de los sistemas comerciales de construcción de techos, como el EPDM, de fieltro, de betún y de metal.¿Qué son los revestimientos de silicona para techos?Los revestimientos de silicona son revestimientos de protección, alto rendimiento e impermeables para techos. Al agregar este revestimiento a un techo con una estructura firme, se puede extender la vida útil del techo existente. La silicona es un componente inorgánico, por lo que mantiene sus propiedades a la intemperie. A su vez, es un material flexible que puede absorber la mayor parte del movimiento normal de un techo a fin de evitar el agrietamiento y la pérdida de sus capacidades de protección.Beneficios de los revestimientos de silicona para techosAdemás de su flexibilidad y su capacidad para extender la vida útil del techo existente, los revestimientos de silicona ofrecen una serie de beneficios. Laura Soder, gerenta sénior de productos líquidos y revestimientos de GAF, explica que los revestimientos de silicona de GAF están diseñados para proteger el techo de filtraciones y ofrecer otras ventajas relacionadas.Protección contra rayos UVEl principal beneficio de los revestimientos de silicona es la protección contra los rayos ultravioleta (UV). "La fórmula de la silicona de GAF incluye dióxido de titanio, lo que ofrece una estabilidad excepcional contra los rayos UV y una reflectancia solar alta", afirma. Esta protección UV ayuda a reducir la temperatura del techo, lo que se traduce en un mejor funcionamiento de las unidades de techo.RentabilidadLos revestimientos de silicona son soluciones rentables que sirven para retrasar el costo de los materiales y la mano de obra necesarios para reemplazar el techo en su totalidad. Funcionan de manera excelente con la mayoría de los techos comerciales y se acoplan especialmente bien con los techos de metal.Restaura el techo existente y extiende su vida útilSoder señala que los revestimientos de silicona se adhieren bien a los techos de metal, lo que los convierte en una excelente opción para extender la vida útil de este tipo de techos. Antes de su aplicación, cepille el óxido ligero o trate específicamente las zonas con más óxido. "Hay muchos techos de metal instalados y, para aquellos que tienen una estructura firme y solo requieren una restauración moderada, se puede agregar con facilidad años de vida útil al techo al revestirlo con silicona", sostiene.Resistente a la humedadLos revestimientos de silicona también son conocidos por su capacidad de resistencia a la humedad. Como la silicona es inorgánica, resiste la degradación en zonas en las que el agua se acumula, lo que la convierte en una opción ideal para áreas con muchas lluvias o nieve.Funciona en climas calurosos y fríosLa silicona tiene un amplio rango de temperaturas en las que se puede aplicar. Al no contener agua, puede aplicarla en temperaturas más bajas que el acrílico y otros revestimientos de techo. Proporciona una capa de impermeabilización monolítica y sin fisuras por encima de los techos de metal existentes. Además, la silicona se contrae y expande junto con el metal en climas fríos y calurosos.En qué se diferencian los revestimientos de silicona de los revestimientos elastoméricosEn comparación con el acrílico y otros revestimientos elastoméricos para techos, la silicona tiene ciertas ventajas.Los acrílicos son revestimientos de protección a base de agua con resistencia a los UV, pero no deben instalarse en lugares donde se acumula el agua, ya que podrían romperse y comenzar a deslaminarse. La silicona es un material que se termina de curar con la humedad, lo que significa que reacciona con la humedad del aire y se seca hasta lograr una película.Soder explica que ambos materiales son flexibles y adecuados para su uso sobre el metal. Pero, si hay agua estancada, el acrílico no es la mejor opción. "Si bien la silicona es más costosa, en general, se degrada a un ritmo mucho más lento que otros revestimientos", sostiene. Ahora bien, una de las desventajas de la silicona en comparación con otros revestimientos elastoméricos para techos es que es resbaladiza cuando está mojada.Recorrido por la instalación y aplicaciónSi bien los revestimientos de silicona pueden extender la vida útil de un techo existente, Soder sostiene que es mejor instalar el revestimiento antes de que finalice la vida útil de la membrana existente.Como las filtraciones suelen ocurrir en las juntas de los techos, puede agregar sellador de silicona en esas zonas. La fórmula de los selladores difiere de la de los revestimientos: en la de los selladores se utilizan otros polímeros de silicona, lo que los vuelve más robustos y pesados. Los selladores de silicona están formulados para las zonas de mucha presión y pueden ayudar a absorber el movimiento en puntos críticos del techo. Funcionan junto a un revestimiento de silicona para la protección del techo.Antes de aplicar cualquier revestimiento, asegúrese de que el techo esté limpio, seco y firme. "Limpio significa libre de contaminantes, polvo, aceites, hojas y otros desechos", explica Soder. Puede usar el concentrado de limpieza de GAF para hidrolavar el techo.Como la silicona se termina de secar con la humedad, el techo debe estar seco antes de aplicarla. Revestir una superficie húmeda puede afectar la adhesión del material y es uno de los errores más grandes que puede cometer en el momento de la instalación. Queremos que el revestimiento comience el proceso de secado por la humedad del aire, no de la humedad en el techo.Cómo aplicar revestimientos de siliconaLa aplicación de un revestimiento de silicona para techos implica cinco pasos:Limpiar los desechos del techo y probar que el revestimiento se adhiera correctamente a la superficie.Asegurarse de que el techo esté firme. Reparar las láminas de metal rotas y reemplazar los sujetadores que falten o estén dañados.Tratar todas las juntas y sujetadores con sellador de silicona, como GAF Silicone Mastic. Se debe aplicar con un cepillo a un grosor de 60 milésimas de pulgada o 1/16 pulgadas mojado.Usar el mismo sellador en los bordes, entradas y drenajes.Por último, aplicar el revestimiento de silicona para techos a todo el techo. Algunos revestimientos, como GAF Unisil Silicone, requieren dos capas, mientras que otros, como GAF High Solids Silicone, necesita solo una.Entender las necesidades de mantenimiento y la durabilidadEl mantenimiento de un revestimiento de silicona para techo es fundamental. Abordar los problemas antes de que se vuelvan más difíciles ayuda a minimizar los costos de las reparaciones y maximizar la vida útil del revestimiento.Como el techo se contrae y expande con el tiempo, se pueden producir algunos problemas con las juntas. Una buena regla de oro es hacer inspeccionar el techo cada seis meses. Aplicar un sellador de silicona puede servir para tratar las zonas con filtraciones o grietas. El sellador de silicona es estable contra los rayos UV y no necesita una capa final, según Soder.Agregar revestimientos de silicona para techos a la caja de herramientasCon tantos beneficios, los revestimientos de silicona para techos deberían ser la primera opción en los proyectos de planificación de restauración del techo. Además, al tener tantas opciones disponibles, puede elegir el tipo que sea mejor para cada tipo de techo en el que trabaje. ¿Tiene más preguntas sobre los revestimientos para techos? Los representantes del servicio técnico de GAF estarán encantados de asistirle con su nuevo proyecto de revestimiento.
By Authors Mark Soto
November 19, 2024