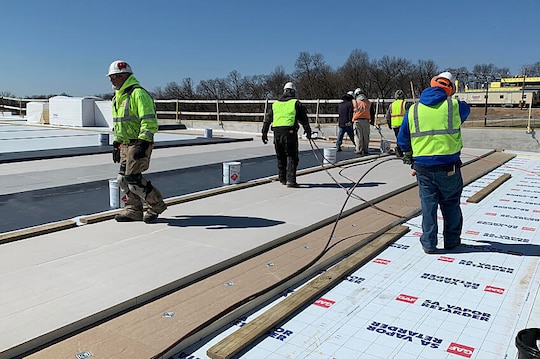
Building Science
Vapor Retarder Frequently Asked Questions
Vapor retarders are increasingly being specified for inclusion in low slope roof assemblies. They can help manage humid air migration from the building interior up to the underside of the roof membrane. Also, they can help limit the amount of moisture migrating from a concrete deck up into the roof assembly. In fact, we offer the GAF SA Vapor Retarder, a self-adhering sheet product, to help reduce this risk. If you are designing a new roof and want to reduce possible moisture risks or are replacing a roof assembly where there's evidence of moisture issues, this article may help you to understand more about the use of vapor retarders.In addition to this article, we also have a Guide to Vapor Retarder Design in Low-slope Roof Systems which describes best installation practices. The guide and this article are intended to address the basics of vapor retarders for designers who want to address moisture migration. Later articles will cover more fundamental considerations.The answers to these frequently asked questions may sometimes repeat key information, and the reader can jump to those questions of most interest. But reading all of the answers will help get a better overall understanding of the function and role of vapor retarders.What is a vapor retarder?It is a material that, depending on its exact specification and correct installation, blocks or slows down the transmission of moisture from one side to the other. Vapor retarders can be coatings, boards with taped joints, or membranes.Looking at this schematic, it is clear that in an actual roof assembly any penetrations have to be sealed otherwise the vapor retarder's function is degraded – more on this later.How does a vapor retarder function?A properly installed vapor retarder at the deck level can help slow down or block the movement of moisture from the building's interior migrating up into the roof roof assembly. Blocking or slowing down the movement of moisture can be part of an effort to lower condensation risks within the roof system during cold winter months.Vapor retarders can also have some degree of moisture permeability. There are different classes of vapor retarding material (I, II, and III) and each class of material allows differing amounts of moisture vapor to pass through the materials via diffusion. The ability to limit vapor movement, but allow some moisture vapor to pass through the material, can be important because it can prevent having trapped moisture within the roof system. Roof membranes are moisture impermeable, so if moisture does get into the assembly, a properly specified vapor retarder with some degree of moisture permeability can allow the moisture to slowly escape downwards.How does a vapor retarder differ from an air barrier?To state the obvious, properly installed air barriers block air, and as a result will also block the movement of humid air, thereby retarding or stopping most moisture movement. So, they can appear to be very similar and sometimes identical. But, the use or application of each is normally different. For more on this, check out this article.A good roof membrane, such as GAF EverGuard TPO will not only block air and moisture but also withstand ordinary wear and tear.A good vapor retarder, such as GAF SA Vapor Retarder, is normally used within a roof assembly to reduce moisture movement. It will have limited or even zero permeability as its primary purpose is to reduce the movement of moisture. While it can act as a temporary roof, it is not intended to be wear- and tear-resistant in the same way as a roof membrane.Is a vapor retarder required?Building codes do not require the installation of a vapor retarder in roof assemblies. A determination as to whether to include a vapor retarder must be made by a design professional. Other answers in this article may help frame what could be considered for such a decision, but a design professional must make the ultimate determination based on the specific conditions at a given project. Basically, a vapor retarder can be specified and correctly detailed in order to manage the migration of moisture vapor to prevent wetting and enable drying within a roof assembly.Does vapor retarder use depend on a building's location?The location has a significant impact on the decision to incorporate a vapor retarder into a roof assembly. The building designer should consider the main direction of moisture drive within the building enclosure. Keep in mind that moisture drive is normally from warm (high vapor pressure) to cold (low vapor pressure).If the building is located in the north, moisture drive is the strongest in the winter. The building interior is usually at a warmer temperature than the exterior. Any interior humid air that reaches the external enclosure layers could cause condensation due to the lower external temperatures. In a roof assembly, a vapor retarder located towards the bottom side of the roof assembly can help reduce or throttle back the migration of water vapor from the interior warm side to the exterior cold top of the roof assembly.For a normal building occupancy and where the building is located in a consistently humid climate, the moisture drive is predominantly towards the interior of the building. In this case, exterior hot humid air that is able to penetrate through the building enclosure can form condensation on interior colder surfaces. Roof membranes are inherently vapor retarders so downward or inward vapor drive is blocked.For buildings with high occupant moisture generation, or that are located somewhere with a mixed vapor drive depending on the season, the roof designer should consider the appropriate roof assembly for the application. If moisture drive from the interior up into the roof assembly could lead to condensation within the roof assembly, then a vapor retarder should be considered.Isn't the roof membrane a vapor retarder? Why do I need another one?Roof membranes are generally vapor impermeable, but to be considered as vapor retarders one has to consider their use. In northern buildings where vapor drive is upwards through the roof assembly, the roof membrane is acting as a barrier to the external weather. It can also be used as an air barrier, preventing interior conditioned air from escaping, but it doesn't prevent interior humid air from moving upwards through the roof assembly. If water vapor is able to migrate upwards towards the roof membrane, then there can be a condensation risk depending on factors such as the exterior temperature and the interior humidity level.Where should a vapor retarder be placed within a roof assembly?The simplest answer to this question is as close to the interior conditioned space as is practically possible. However, always check that local fire codes allow for self-adhering membranes applied directly to steel decks. In many cases it is necessary to first install a gypsum or cementitious board over a steel deck which is then used as a substrate for the adhered vapor retarder. Always check with the roof system designer to make sure that a proposed system meets all necessary codes.Alternatively, the vapor retarder could be applied to the topside of the first layer of insulation, but in such a case, the designer would need to confirm that the dew point would be above the vapor retarder.Can I use black poly (e.g. Visqueen) as a vapor retarder?Black poly sheet, technically 6 mil polyethylene, is often used as a vapor retarder in residential crawl spaces. However, its use in roof systems is generally not recommended for several reasons:It does not self-seal around fasteners that penetrate through it. Vapor retarders such as GAF SA Vapor Retarder are designed to meet a self-seal test described in ASTM D1970.Polyethylene is notoriously difficult to adhere to, which makes flashing and sealing around penetrations very difficult and unlikely to be durable.6 mil polyethylene is essentially impermeable, which means that any leak in the roof covering will let in water that can't escape. Also, if some water has been present when the roof was closed up, from dew or light rain during the previous night, it will not be able to escape. Properly specified vapor retarders have some degree of permeability that will allow for migration of water from within a roof assembly downwards. The only exception to this may be for a building with a very high interior humidity when it might be advisable to have a vapor retarder with essentially no permeabilityMy building is in the north, so do I automatically need a vapor retarder?No, a roof designer needs to evaluate the risk of condensation occurring. The building use, the type of building, and the roof assembly design are important considerations. An evaluation of condensation risk asks questions including:What is the humidity level likely to be in the building? Office buildings can be expected to have lower levels versus buildings with restaurants or indoor pools.If activities within the building could generate high humidity levels, has the HVAC system been designed to reduce the levels with make-up air?What is the building's location and what are the coldest exterior temperatures that could be expected?Will the roof assembly inhibit air flow without the use of a vapor retarder? Some roof assemblies, particularly those that have adhered layers, are more restrictive of air flow than others.Once interior humidity levels have been estimated and outdoor cold temperatures known, then the building designer can calculate where the dew point will be in the roof assembly. If the designer specifies a vapor retarder, it should always be located below the dew point.How should a vapor retarder be tied in, flashed to penetrations, etc?To be successful, penetrations through the vapor retarder need to be flashed and air tight. Also, the edges need to be terminated to the walls. Care has to be taken to ensure that interior air cannot readily move past the vapor retarder and up into the roof assembly or a parapet wall. The GAF Guide to Vapor Retarder Design in Low-slope Roof Systems provides system details to help guide good design.Should a vapor retarder be used with a concrete deck?In new construction, it can be difficult to ascertain when concrete decks are sufficiently dry to allow the roof assembly to be installed. If significant levels of moisture are present in the concrete deck after the roof is closed up, then problems can arise.For more on the topic of moisture in concrete roof decks see this article by my colleague James Kirby. Briefly, as advised by industry groups such as the Midwest Roofing Contractors Association (MRCA), the use of a vapor retarder over a concrete deck will limit moisture passing through to the roof assembly.In re-roofing situations over concrete decks, there is usually less concern about moisture being present within the concrete deck, providing that there have been no leaks. However, if the roof was originally installed with minimal insulation, it could be that the concrete contains significant amounts of moisture due to condensation, depending on the local climate. Also, any precipitation during reroofing could allow a concrete deck to absorb quantities of water. If there is any concern about moisture in an existing concrete deck, a vapor retarder should be considered.In new construction, is there a concern about moisture from a concrete floor or foundation?Yes, there can be, depending on location and other factors. In some big box construction, when the building has been closed up quickly after a floor slab was poured, condensation issues have occurred during the first year of occupancy. This is related to high interior humidity as the concrete dries out for months during and after construction. Concrete floors and foundations can take a long time to dry and as a result interior moisture levels can be high enough that condensation has been known to occur in climate zones 3 and 4.Building designers and architects of such buildings often include a vapor retarder in the roof assembly in order to reduce condensation risks during construction, after the building is closed up, and for up to 12 months later.What about where the vapor retarder meets the edge of the roof?Sealing and termination of vapor retarders around the perimeter is difficult. Building designers should recognize that the goal of a vapor retarder is to block the movement of interior humid air up into a roof assembly and to controllably allow for some vapor permeability so that moisture that does enter the roof assembly can migrate down into the building. The GAF Guide to Vapor Retarder Design in Low-slope Roof Systems provides edge termination details to help guide good design.I often see dew point and vapor retarders being discussed together. Why?It's important to make sure that a vapor retarder is installed below where the dew point in the roof assembly is calculated to be. The vapor retarder will then reduce the likelihood of moisture reaching that position and forming condensation during cold periods. Calculation of the dew point takes into account the expected interior humidity levels and the possible exterior temperatures.What is condensation risk and should I always include a vapor retarder?Moisture is well known to lead to problems with respect to the durability of a building enclosure. The risk of condensation within a roof assembly should be assessed by a design professional. The analysis should consider factors including climate and building use. It is important to recognize that there often is not a definitive yes/no answer to the question as to whether a vapor retarder is needed.The colder the climate, the higher the risk of condensation within the roof assembly. So, buildings located in northerly regions will generally have a higher risk of condensation forming in the building enclosure.The higher the anticipated interior moisture load, the higher the risk. Office buildings occupied during daytime only are likely to have a lower risk versus a building that includes a swimming pool. A building closed up during construction while a concrete slab floor is still drying will likely have a higher risk.The roof assembly design is also a factor. High wind events can cause mechanically attached single-ply membranes to billow which causes air to be drawn up into the assembly, which can increase the risk of condensation. To minimize condensation risk, roof designers should first consider adhering the roof membrane and upper layer of insulation, making it harder for interior air and moisture to be drawn up into the assembly. If a cover board is being used, it should also be adhered.ConclusionsVapor retarders can be used to reduce the movement of vapor within a roof assembly. They need to be positioned as low as practical within the assembly and any penetrations should be sealed. Vapor retarders in the roof assembly may be beneficial in buildings with large temperature differences from interior to exterior throughout the year, and occupancies with higher than normal interior moisture levels, either from use or during construction.Important ConsiderationsThe purpose of this article is to provide some background information and design considerations for roofing assemblies using vapor retarders. GAF manufactures and sells roof materials but is not responsible for building design and construction. Design responsibility remains with the architect, engineer, roofing contractor, or owner. This information should not be construed as being all-inclusive, nor should it be considered as a substitute for good application practices. Please consult your design professional for more information.
By Authors Thomas J Taylor
June 01, 2021